Abstract
Several segments across the economy – industry, commerce, agribusiness – are increasingly looking to deploy technologies that have been called Industry 4.0. These technologies enable a huge leap forward in productivity, efficiency, integrated management, better processes, and a whole range of tools enhancing the future of production.
To introduce these technologies, there is one essential factor: connectivity. This connectivity can be obtained in several ways, but the most attractive are the increasingly affordable 5G Private Networks that deliver broadband with low delay, mobility, stability, and security.
This article describes 5G Private Networks, their technical characteristics, deployments, and some of the multiple use-cases it has enabled.
Introduction
Industries and businesses are undergoing an unprecedented digital transformation driven by cloud-native applications and services, internet of things (IoT), data analytics, artificial intelligence, augmented reality and blockchain. While the degree of implementation of these technologies varies by segment or vertical, one common factor holds true across the board – the need for connectivity and mobility on fast and stable networks.
A 5G Private Network is a corporate network that furnishes communication connections for users belonging to a private organization, while offering application-specific services tailored to the needs of each business. For industrial applications, the ability to deploy mobile networks to meet reliability, latency and security requirements for critical applications is crucial for the new wave of cyber-physical systems known as Industry 4.0.
Different companies have stringent requirements that cannot be met by using traditional Ethernet or Wi-Fi networks. Networks built with wired Ethernet are cost-effective and stable in terms of performance and quality but limited in mobility while their maintenance and scaling are complex tasks. Wi-Fi networks are easy to build and operate, but in terms of communication performance – such as connectivity distance, latency, mobility, and security – they do not meet the requirements of corporate digital transformation.
Considering these existing types of operation, a mobile communication network that can overcome the limitations of traditional Ethernet and Wi-Fi networks gives 5G Private Networks a competitive edge to optimize their business.
5G Private Networks may be deployed across a wide range of modes and is much more flexible than previous mobile communication generations. 5G frequencies allocations to set up private networks have been introduced and standardized by several countries in recent years. In Brazil, this process is still underway at Anatel but is due to conclude soon, probably with the allocation of 100 Mhz bandwidth in 3700-3800 MHz.The new 5G frequencies for use by private networks are described in different ways such as unlicensed, private, local or shared frequencies while CBRS (Citizens Broadband Radio Service) is a prime example now being used in the US.
5G technology use cases (URLLC, eMBB, mMTC) are driving innovation in smart factories, transportation, logistics, smart hospitals, and so forth.
Network slicing, which is one of the most significant features of 5G, creates a range of logical networks addressing the needs of Industry 4.0 technologies, thus enabling the creation and delivery of optimized services for private networks.
Virtualization and cloudification enable 5G networks to use software in hardware-agnostic environments. Mobile networks deployed with software – which are not tied to dedicated hardware equipment – help private network customers lower costs while boosting efficiency for their deployments and operations.
The purpose of this document is to show that 5G private networks can achieve excellence in their performance levels. This article therefore explores the ways in which companies and organizations may deploy and operate private networks and introduce innovative 5G technologies for the high-level performance that private networks require.
5G Private Network Architectures
A public mobile network is typically deployed and operated by public mobile network operators (MNOs) on licensed spectrums. In the case of a private network, however, ownership of deployment and operation may be transferred from MNOs to the company deploying the network and may be created even in unlicensed spectrums independently of public networks.
Deployments of 5G Private Networks may divided into two categories:
- Independent network – autonomous private networks fully isolated from a public network;
- Dependent Network – Private networks deployed in conjunction with a public network, using the following:
Private network sharing RAN;
Private network sharing RAN and control plane;
Private network deployed inside a Public Network.
Independent Network
This scenario envisages a private network deployed as standalone network that is physically and logically isolated from a public network. In addition, an autonomous private network may be completely isolated from the public network using local 5G spectrum instead of an MNO’s licensed spectrum. If specific use cases are needed or there are regulations to be complied with, licensed spectrums may be used too.
All network functions such as gNB, control plane and user plane are deployed on company premises as Figure 1 shows. Once the company has its independent 5G network with all functions at its disposal, it can apply the relevant innovative 5G technologies and it is not restricted to traditional Ethernet or wireless LAN solutions.
The typical advantages of an autonomous private network are summarized below.
- Security: An independent network completely separate from the public network ensures security and privacy while preventing leakage of internal traffic and subscriber data.
- Ultra-low latency: All network functions are located inside the company, so network delay between device and application server is minimal, enabling ultra-low latency services such as motion control applications.
- Customized quality of services: Network settings for private networks may be customized to meet corporate application requirements, unlike a public network’s default configuration. The configuration includes all parameters that may affect data rate, reliability, latency, as well as uplink/downlink frame structure.
- Autonomy: the nature of an autonomous private network ensures its independent operation even if there are faults (e.g. network downtime and degraded performance) on the MNO’s public 5G network.
However, acquiring and deploying an entire 5G network can be a challenging solution for companies lacking sufficient telecommunication technology experience and know-how. That’s why it’s important to select a partner that has the knowledge, experience, and support service to effectively implement and customize a 5G network for your business.
Dependent Network
1.Private network with RAN sharing
In this scenario, a private network shares part of the RAN – especially the gNB – with the public network, while other network functions are deployed across the company and remain separate from the public network, as shown in Figure 2.
This deployment scenario is further divided into two sub-scenarios, depending on whether the private network uses local private spectrum or the operator’s licensed spectrum, which is shared with the public network.
This scenario is similar to an autonomous private network, except that private and public networks share parts of the RAN in this scenario. Data traffic from the private network – Figure 2’s private slice – is delivered to the 5G private user plane in the company, while data traffic from the public network – Figure 2’s public slice – is delivered to the 5G core user plane in the mobile operator’s public network.
Here, the private 5G core control plane is also deployed in the company, so private network operation and subscriber data stay inside the company, so they are not shared. Therefore, the private network’s security and privacy can still be guaranteed, even though parts of the RAN are not completely separate.
Additionally, in this model, all network functions are located on the company’s premises, thus delivering ultra-low latency communications. In this scenario, however, RAN failures may cause private network faults that disrupt installed services and use cases.
2.Private Network sharing Control Plane and RAN
In this scenario, private and public networks share the same RAN, while the network is controlled by the public network. The general architecture of private and public networks and their components are shown in Figure 3.
Control plane functions for a private network’s subscribers, such as authentication or mobility, are performed in the mobile operator’s network control domain, together with control of public network subscribers. In other words, devices on the private network are, by definition, MNO’s public network subscribers. This allows devices on the private network to directly access services on the public network and ensures continuity of service (roaming when devices on the private network move between private and public networks, and vice versa). However, interworking with the public network leaves security concerns, such as operational and subscription data from private network devices being stored on a mobile operator’s server rather than stored on company premises.
Data traffic between private and public networks may be separated using technologies defined by 3GPP, such as network slicing. Networks are separated using different network slice identifiers. Data traffic from the private network is delivered to the company’s 5G core user plane with its identifier, while data traffic from the public network is delivered to the main 5G user’s plane on the mobile operator’s public network, as shown in Figure 3.
This scenario also ensures minimal latency by introducing multi-access edge computing (MEC) on the company’s premises. The ultra-low latency communication between the UE-gNB-5GC-MEC enables the company to use ultra-reliable, low-latency communication (URLLC) applications such as autonomous driving and real-time robot control.
3.Private Network Implemented in Public Network
Figure 4 shows a private network that is fully hosted on the public network. In this scenario, only the gNB is deployed in the company whereas all other network functions are shared with the public network. All data traffic from private and public networks flows through the public network located outside the company, but traffic from each network may be virtually independent of each other due to separation using end-to-end network slicing.
In these scenarios, the mobile operator will have full control over security and privacy as the deployment scenario is completely dependent on the public network and the failure of the public network directly leads to the failure of the private network. Additionally, using low latency services such as URLLC may be difficult. Since the MEC is also restricted to the mobile operator’s network, the network’s round-trip time (RTT) may be much longer depending on the distance between the company and the edge cloud of operator where MEC is located.
This deployment scenario is hosted on the operator’s network, so this solution incurs monthly operating expense for the company, unlike other scenarios in which network functions are built in-house.
But an advantage is that service continuity may be ensured through roaming when devices move between private and public networks.
Use Cases for 5G Private Networks
There are numerous possible use cases when a 5G private network is built in a company. Each of the different business verticals has specific needs, which are added to a wide range of applications on the market that may be of use for all companies.
Here are a few examples of 5G Private Networks used for the main verticals:
a) Intelligent Factory
Manufacturing industry is a segment in which the advantages of a 5G Private Network are clearly perceived. When Industry 4.0 technologies are deployed through 5G connectivity, materially higher efficiency is expected for the manufacturing sector as well as improvements across all production related areas, including planning, material control, line control, production capacity, inventory management, dispatch, security, training, amid many other applications.
5G technology meets manufacturing segments’ high standard requirements that could not be covered by existing technologies. In the early stage of 5G technology, the enhanced mobile service based on broadband (eMBB) will be introduced; later, the URLLC resource, which is essential for special applications, will be applied to mission-critical use cases such as self-guided vehicles; then the MMTC resource provides the technologies needed to deploy multiple use cases involving IoT devices in large numbers (massive IoT).
The ability to create priority services within the network will be applicable by providing adequate network slices for the various network services required within the factory. Figure 5 illustrates use cases that the smart factory supports.
Some solutions made possible by the introduction of 5G private networks for automation solutions in a manufacturing environment:
- Creation of virtual stores and showrooms with AR/VR;
- Integration of warehouses with the chain of stores in the chain;
- Inventory control;
- Internal autonomous transport;
- Surveillance and security.
b) Transportation and Logistics
5G technology creates a new private communications framework for the transportation and logistics vertical, providing real-time tracking and enhanced visibility of all assets, cargo inventories and packages on the network.
5G technology is suitable for use cases that require much higher density than existing IoT technologies such as Sigfox, LoRa and NB-IoT.It is possible to implement a more efficient and optimized supply chain management, using ML and AI technologies.
In the case of transport and logistics, all related data is collected by the 5G network through sensors that provide greater availability and connectivity. The analysis of this data, in conjunction with ML/AI technology, provides the necessary insights. In addition, 5G can be used to control self-driving vehicles to improve productivity and ensure safety at the same time.
New services and business models may be supported with multiple sensors embedded in highways, airports, railways and for communication with smart vehicles:
• Autonomous vehicles
• Connected bus stops
• Connected trucks
• Connected cars
• Remotely controlled heavy machinery
5G technology brings:
• Sustainability
• Security
• Fleet monitoring
• Navigation and augmented reality
• Ecosystem scale and diversity
Target Users:
• Automotive
• Security and insurance
• Transport and infrastructure companies
• Administration/governments
Additional use cases that can be enhanced by 5G technology for transport and logistics are shown in Figure 6.
These and many other applications are connected via 5G Private Networks, either conventionally or through open disaggregated architecture – OpenRAN. This is the typical scenario in which a Private Network has an application for connectivity controlled by the company’s management, meeting all demands without restrictions or cybersecurity difficulties.
c) Smart City
5G technology adds new impetus to the development of smart cities. The 5G network is an ideal infrastructure for an integrated urban platform.
Before 5G technology, establishing a massive IoT environment was simply not feasible, with only IoT sensors operated in a few local areas. With 5G technology, a range of sensor networks covering the entire city can operate based on a single massive IoT technology (MMTC). All of a city’s surveillance cameras and sensors for temperature, humidity, and pollution as well as all its traffic signs may be connected to an urban control center via 5G networks. This poses the possibility of managing the entire city as one single organism. Therefore, 5G technology can enhance overall urban organization and security.
A 5G network is a large infrastructure that can evolve into an AI system based on big data that analyzes data such as an intelligent video surveillance network, road traffic network, main facility monitoring network, emergency services and meteorological/environmental monitoring network. Additional use cases for a smart city are shown in Figure 7.
A municipal administration does not necessarily have to use commercial 5G network infrastructure to build a network like the one mentioned above. Private Networks may be used for pilot projects and then deployment may be scaled up based on these experiences. Private Networks are easy to install and operate using both conventional infrastructure and OpenRAN infrastructure and architecture.
d) Medical service
5G technology can break time and space constraints affecting contacts between doctors and patients. Remote surgical operations will require high bandwidth for HD/4K video and minimal delay (<5ms) to properly manipulation artificial surgical arms or for real-time communication between surgeons and remote medical teams.
Surgical training may be delivered through AR/VR goggles for medical training. In addition, doctors can diagnose a patient far from a hospital through real-time high-definition video on mobile networks.
Sensors and wearable devices – specially designed for medical use – may be used more widely in medicine with 5G characteristics such as high reliability and low delay. Additionally, these sensors provide medical AI with massive amounts of data to be queried and analyzed to constantly enhance the overall quality of medical services. Figure 8 shows expected use cases for medical services on 5G private networks.
These and multiple other applications using remote sensors and actuators connected via 5G networks are called smart hospitals. A smart hospital focuses on automated processes using an interconnected environment based on IoMT (Internet of Medical Things), creating a connection between patients, doctors/nurses and devices.
Connections may use conventional or OpenRAN 5G networks. This is the typical scenario where a Private Network has an application, enabling connectivity under the control of a hospital administration and meeting all demands free of cybersecurity restrictions or difficulties.
Some examples of IoMT devices:
- A device developed by Apple uses its built-in IMU (inertial measurement unit) to detect a patient fall. It may be used to measure tremors related to nervous system disorders such as Parkinson’s disease.
- Another example is a pill with a tiny sensor, which transmits information to an adhesive patch on a patient’s arm. When the pill is taken, the patch receives the information and relays it to a cell phone monitored by the doctor, thus ensuring that the medication is being taken on time and in the correct dosage.
- A partnership between Novartis and Google created a contact lens for diabetics, which measures blood sugar in tear fluid. These lenses provide continuous blood sugar fluctuation data and inform patients in real time.
- Other examples of the use of IoMT: blood tests capable of predicting premature births; tubes that may be swallowed to facilitate screening for intestinal diseases; personalized cancer vaccines that can reprogram the body to attack only cancer cells; voice assistants in medical clinics, among many other uses.
Use cases in hospitals are extensive:
- Smart Operating Rooms
- Smart medication
- Digital biomarkers
- I – robotics
- Aerial thermal scanners
- Dimensional scanning and printing
- Virtual planning, simulation & navigation guided surgery
- Mobile and smartphone applications (apps)
- E-learning
- Tele-dentistry
- Voice assistants
- Ambient assisted living
- Adverse drug reaction detection
Worldwide demand for smart hospital-type applications and projects has been growing in recent months, and some practical implementations have already been reported, including here in Brazil. These projects plan for 5G Private Networks installed in hospital environments with subsequent gradual introduction of use cases, exploring high upload/download speeds and low delay time.
Fig. 10 below shows consultants’ research results with forecasts for this vertical:
e) Energy Networks
The conventional architecture of electrical distribution networks poses several obstacles that do not allow implementation of new technologies, such as those expected for so-called Industry 4.0. This architecture has the following characteristics:
- Limited data collection;
- Inefficient communication networks;
- Slow remote operation;
- Focus on protection after failures;
- Vulnerability to vandalism and natural disasters.
The Smart Grid poses an unprecedented opportunity to move the energy industry into a new era of reliability, availability and efficiency that will contribute to economic progress and environmental improvement. Benefits associated with Smart Grids include:
- More efficient electricity transmission;
- Faster restoration of electricity after power outages;
- Reduced OPEX costs for utilities and, ultimately, lower energy costs for consumers too;
- Reduced peak demand, which will also help to lower electricity tariffs;
- More integrated large-scale renewable energy systems;
- Better integration of energy generating systems, including renewable energy systems;
- Enhanced security.
Currently, a power outage can cause a domino effect – a series of failures that can affect banking, communications, traffic, and security. A smart grid will add resilience to the electrical power system so it will be better prepared to deal with emergencies such as storms, fires, solar flares, and vandalism. Due to its interactive capability, the Smart Grid will allow automatic rerouting when equipment fails, or outages occur. This will minimize interruptions and effects when they happen. When a power outage occurs, Smart Grid technologies detect and isolate outages to contain them before they become full-scale blackouts.
New technologies will also help ensure that electricity recovers quickly and strategically after an emergency – by prioritizing electricity for emergency services, for example. In addition, Smart Grids will make more use of private energy generators to produce energy when utilities need it. By combining these “distributed generation” resources, a community could keep its core infrastructure running during emergencies.
Smart Grids are not just about utilities and technologies; they are about providing the information and tools needed to make choices about energy use. “Smart meters” and other mechanisms will allow consumers to see how much electricity they are using, when they are using it and how much it will cost. Combined with real-time pricing, this will enable savings by using less energy when electricity is more expensive.
Energy & Telecom Convergence in Smart Grids:
- Accelerating digital transformation of networks and neutralizing the Carbon Footprint: ecology and public image;
- Intelligent detection, measurement, control, and supervision: focus on quality and prevention;
- Sustainable power generation, with higher energy efficiency: fewer interruptions, greater profitability;
- Quick access to real-time energy generation & consumer data: fast reconfiguration.
Technologies involved in Smart Grids:
- New technologies integrated into the Network: IoT, Clouds, AI, ML, 5G, drones, robots…;
- Wireless consumption meters: lower costs;
- Automated intelligent electrical grid inspection: hours vs days;
- Network protected against chain reaction: higher quality service;
- Augmented Reality and Remote Assistance via Video Conferencing: maintenance facilitated.
Use Case: Load Control
- Precise instantaneous load control;
- Constant interaction between network and sources/loads;
- Instant supply equilibrium;
- Avoids mass power outages;
- Minimizes network losses and impact on business and home consumption;
- Consists of service terminals, demand response terminals, a communication network, and a master station system;
- Connection via local network and then to the demand response system’s master station via communication network (e.g. 4G/5G).
Use Case: Differential Protection
- Solution for problems caused by connecting distributed power sources and fluctuations in distribution grid load;
- Compares current across two or more differential protection terminals at the same time. If the difference exceeds its limit, the terminal detects a fault and disconnects the differential protection switches, thus enabling accurate localization and isolation of network faults;
- The distribution management system dynamically analyzes the topology of the entire distribution network and transmits topology alteration data to each distribution or feeder terminal (DTU or FTU);
- The service is fast, dynamically adaptable, and reliable for fault detection purposes.
Use Case: Consumption Control
- Automatically collecting electricity consumption data, monitoring metering anomalies, monitoring power quality, analysis, and management of electricity consumption;
- System consisting of master stations, remote and local channels, concentrators, and collectors/meters;
- The electricity consumption data collection system is virtually divided into master station layer, communication channel layer and collection device layer;
- Wired and wireless communication channels, such as fiber optic private network, wireless public network, private wireless network.
Use Case: Mobile Inspection
- Current networks: inspection is manual or with robots/drones connected via 3G, with most inspection videos held locally at the station;
- Smart Grids: real time transmission to remote monitoring center via 4G/5G;
- Remote monitoring: collecting and identifying photos and videos of power distribution equipment to gather operating and working status data;
- Improved operating and maintenance efficiency;
- Lower labor costs
Smart meters for homes and industries
Smart meters work with a smart energy monitor that you may place anywhere in your home. This will enable you to see how much energy you are using and show how much it is costing you.
Smart meters have been introduced in many homes around the world using existing telecommunications infrastructure to manage, send and monitor data. However, with the introduction of 5G, these services will be much more accurate, so more data may be sent and received more frequently, resulting in much more detail for both consumers and service providers.
With 5G data will reach higher levels of detail, thus enabling consumers to visualize the individual items in their home that are using most electricity and show the specific times of day when we incur the highest costs.
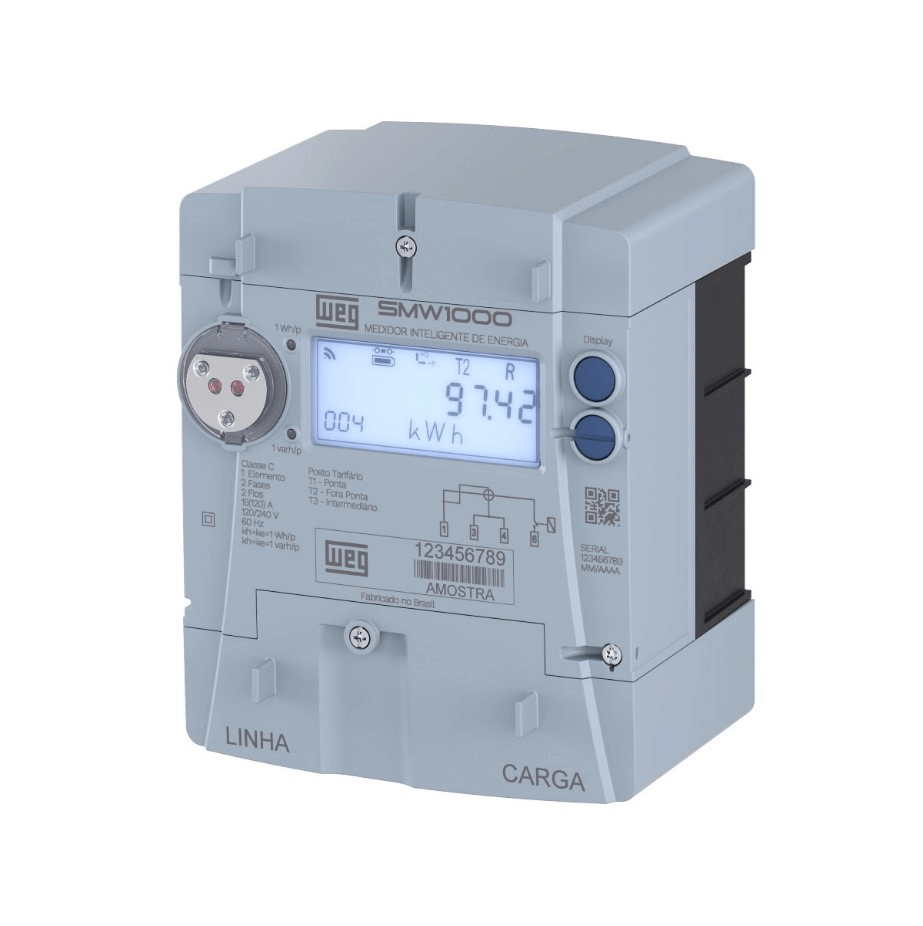
Challenges of 5G Private Networks
As mentioned above, 5G Private Networks may be used for a number of business verticals, including smart cities, factories, logistics, hospitals, and many others. But this implementation of cellular coverage alone does not meet the various needs of business. A dedicated solution – which addresses the unique characteristics of each vertical – is required, which may pose challenges for the design and deployment of these networks.
The coverage of 5G Private Networks depends on the type of business environment of a company. Compared to the conventional cellular network environment, the demand for in-house solutions is much higher and demand for unique networks is growing too. Therefore, 5G Private Networks must support a wide range of RAN products that can work in any corporate environment.
Together with broad RAN coverage, Private Networks have to be compact in both size and consumption. The capacity required of a 5G Private Network is normally less than for a macro network. In addition, its equipment has to be small because it must be installed in a confined space.
Another challenge comes from the nature of the 5G Private Network. This is a dedicated network for a company that requires simple unhindered operation and generates updated reports that enable even an inexperienced operator to manage it.
Spectrum Analysis
Anatel is studying not only an offer of more spectrum for mobile services, but also how it intends to do so. The proposal is to provide a “frequency menu” for companies in both 4G and 5G, but not withdrawing bands already used for commercial networks. The menu will be divided by regions across the spectrum since the superintendent states that “different applications may require different frequencies”.
The strategy is not withdrawing frequency bands from commercial networks but adding more non-IMT or IMT bands that have restrictions that would hinder ubiquitous application. In other words, even there is spectrum outside the 3GPP standard, the band could be made available for certain indoor applications, which would reduce the risk of any type of interference in other external services.
So the currently available bands would look like this:
- 225-270 MHz
- 1,487-1,517 MHz
- 2,390-2,400 MHz
- 2,485-2,495 MHz
- 3,700-3,800 MHz
- 27.5-27.9 GHz
Other bands that may be on the menu but are still in the final stages before release are 410-415 MHz and 420-425 MHz.
Anatel is now working to offer more capacity for mobile services: the refarming of the 850 MHz band, specifically to free up continuous blocks in the band, and the addition of viable spectrum at 800 MHz (band 26 of 3GPP). The proposal also offers the 4.9 GHz spectrum (4,800-4,960 MHz). There is also an intention to offer the 1.5 GHz frequency, of which 1,427-1,487 MHz would be for operators and 1,487-1,517 MHz for private networks.
Anatel provides protection for mobile satellite service (MSS) operating above 1,525 MHz. This concern was raised by operators in a public consultation for the draft resolution that approved the Plan for Assignment, Allocation and Distribution of Frequency Bands in Brazil (PDFF), in January 2021, since the multi-destination of the L band (1,492-1,560.5 MHz) would enter the MSS (1,518-1,560.5 MHz).